You might think that metals don’t like to burn. Quite the opposite – they love to burn. You just need to help them get in touch with enough oxygen.
We don’t see frying pans catching fire
We use metals every day, and our experience tells us that metals do not burn. We cook in metal cookware directly over flame, and we do not see our pot igniting. It may come as a surprise, therefore, to learn that metals love to burn. And that they burn with high temperatures and more brightly than regular flames that you usually see. Hence, igniting a metal like iron is a great entertainment for kids (and an ideal material for edutainment).
So, where is the catch? First, we need to define burning. In this case we mean a chemical reaction (called oxidation) that needs a material to burn (metals in this story), a supply of oxygen (air in this case), and a source of heat to initiate the chemical reaction. The result of burning in this case is a metal oxide – a molecule that combines metal and oxygen atoms. Still, if we take a nail and try to ignite it with a lighter, nothing happens (although the nail will eventually get too hot to hold). Something else is preventing a chunk of metal, like iron, to easily catch on fire.
There are several obstacles to this burning reaction:
Oxygen supply: Burning requires a constant supply of oxygen and metal, which is not easy to do if the metal atoms are buried below the metal surface. A reaction can happen on the metal surface, but that’s essentially it. The metal gets covered by a layer of metal oxide, which acts as a barrier for further supply of oxygen from air. Actually, in industry this is done on purpose to protect metals from corrosion. Also, since metals don't vaporize easily, you can’t produce a gas mixture of metals and oxygen above the surface. A gas mixture would have many atoms ready to react. For example, in candles, evaporation of candle wax enables them to burn steadily.
High ignition temperature: Metal atoms are strongly bounded to each other. Therefore, reacting atoms need a strong kick (i.e., high temperature) to get away from other metal atoms and become ready for a reaction with oxygen.
Overcoming loss of heat: Metals are good conductors of heat (i.e., vibrations are easily transferred far away along the metal structure). This means that if you try to burn a part of a metal object, the heat will flow away from that spot. By the way, that’s why “the thicker the better” is a general measure for a good cookware.
It is always about tricks
Still, even though it looks like you would need a special high temperature oven to burn iron, there is one trick that we can play. If we get our hands on tiny iron strings, or iron dust, we can overcome all the above-mentioned obstacles. The reason why this works can be explained with the following example.
Imagine a wire that has a square cross section. If the sides of this square are of length L, then the circumference is 4*L, while the area of cross section is L*L. Notice that burning will depend on the wire’s surface that itself depends on the 4*L circumference. On the other hand, the thermal conductivity (the ability to transfer heat energy along the wire) depends on the area of wire’s cross section L*L.
If we now cut this wire into four square wires of equal cross section, each new smaller wire will have the circumference of 4* L/2=2*L and the cross section area of (L/2)*(L/2)=L*L/4. This way we increased the overall surface exposed to oxygen because all four wires combined now have the surface 4*(2*L)=8*L. In other words, reducing the wire’s thickness by the factor of X=2 increased the exposed surface X times and reduced the cross section area available to the heat transfer X*X times.
If we repeat this process again for each small wire, the scaling factor would be X=4. This increases the overall exposed surface by the factor of X=4 and decreases the cross section area of each wire by the factor of X*X=16. You can see now how transforming a chunk of metal into tiny metal threads helps us to avoid all three key obstacles to burning: the amount of metal exposed to oxygen is significantly increased, while the metal’s ability to efficiently transfer heat is suppressed so much that we can easily increase its temperature.
This kind of behavior in nature is known as a scaling law and it is ubiquitous in biology, physics, economy, and nature in general.
For example, scaling properties of tubes that bring oxygen directly to the tissue within insects’ body dictates how big insects can grow. These tubes, called tracheae, are highly branched, which enables them to provide a large surface for respiratory gas exchange. But the volume of tubes increases faster than their surface when the insect’s body is increased (volume scales with the cube of the insect’s size, but the surface scales with only the square). You can see this scaling property in our discussion on wires. In the end, insects hit a size limit where their body becomes an inefficient hollow system of tubes.
Let’s burn something!
OK, now that we learned about the trick, let’s start burning some iron! But let’s be educational and start with something less dramatic first. Before jumping to playing with actual fire, we can demonstrate that iron oxidation is a highly exothermic reaction. This means that formation of rust, which is a form of iron oxide molecule, releases heat.

The experiment is quite simple:
Take a fine grade steel wool (you can find it in a local hardware store) and soke it in vinegar for a minute or two.
When you notice the first small bubbles forming (this is hydrogen), take it out and squeeze out as much vinegar as you can.
Wait a little bit and you will notice that the wool starts to warm up. The temperature increase depends on the wool grade and how much air comes in contact with iron.
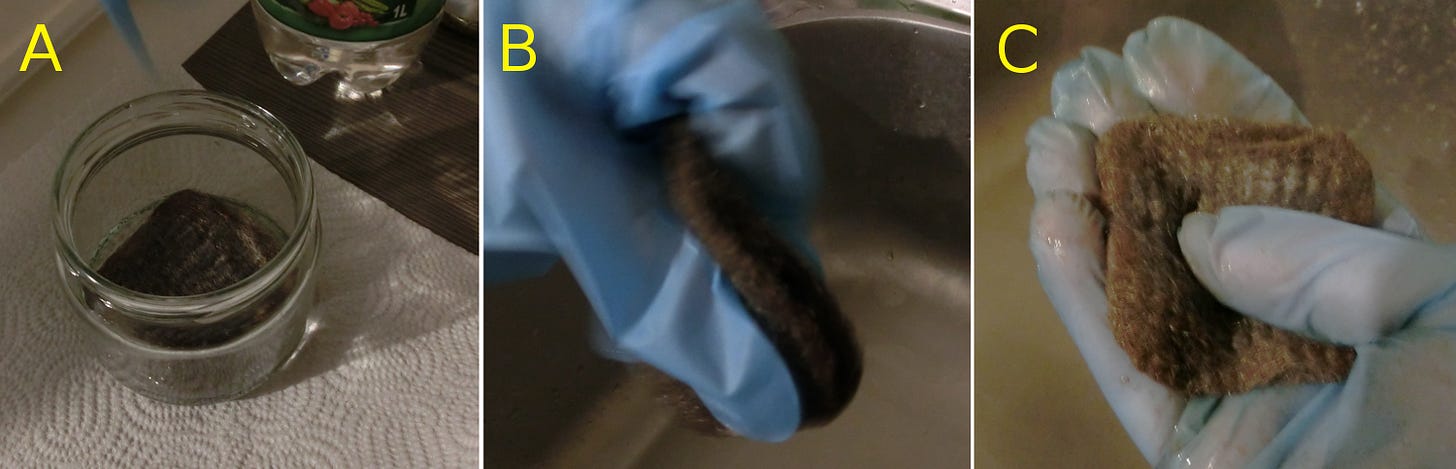
What happens here is a two-step process. First, the steel wool is a bundle of tiny steel (i.e., iron) threads that are covered by a fine layer of oil coating. This oil is added during manufacturing to protect the steel from the reaction we want to see in this experiment – oxidation. Vinegar contains the acetic acid that can remove this protective coating. Once the coating is removed, we get rid of vinegar and expose the iron to oxygen in the air. Iron and oxygen start to from Fe₂O₃ molecules that are the basic component of rust.
You will see this rust as a brown substance appearing on the wool. The important message here is that you can notice how the wool gets warmer in your hand. You can also wrap the wool around a thermometer and measure the temperature increase. If you are a teacher or enthusiastic parent, there are many interesting details that you can explore further in this experiment.
Let’s burn something now for real!
And finally, we are ready to ignite some iron! So, what have we learned so far? First, using very thin iron threads increases the reaction surface between iron and oxygen, while at the same time reduces the ability of iron to remove heat away from the burning area. Second, oxidation releases heat of its own, which means that very efficient iron oxidation might release so much heat that the reaction would keep supporting itself once we start it.

All you need now is a fine grade steel wool. You can ignite it with a match or by touching it with 9V battery. The wool threads are so tiny that the electric current heats them up so much that they ignite. This burning iron reaches temperatures of about 700ºC, which is so hot that burning threads ignite nearby threads. This results in a very pretty scene of burning process slowly traveling all over the wool.

The iron oxide molecule formed by oxidation in this burning is different from rusting. Here the reaction produces mostly Fe₃O₄, known as magnetite (other versions of iron oxides will also appear, depending on how much oxygen was available at the iron surface). It is interesting to observe how the wool becomes heavier after burning, unlike what we experience in typical burning of organic material. Burning of iron adds oxygen atoms into the iron oxide that stays on the steel wool and makes it heavier. When we burn wood the opposite happens – burning releases water and carbon dioxide gasses that evaporate away and the reaming wood is transformed into a lightweight ash.
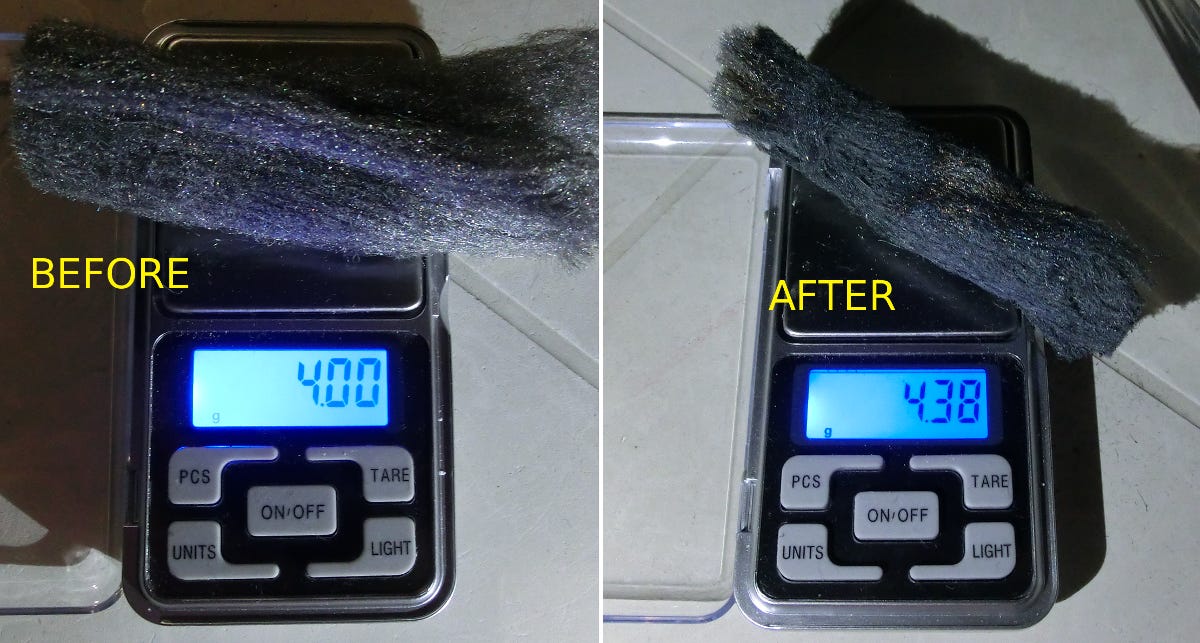
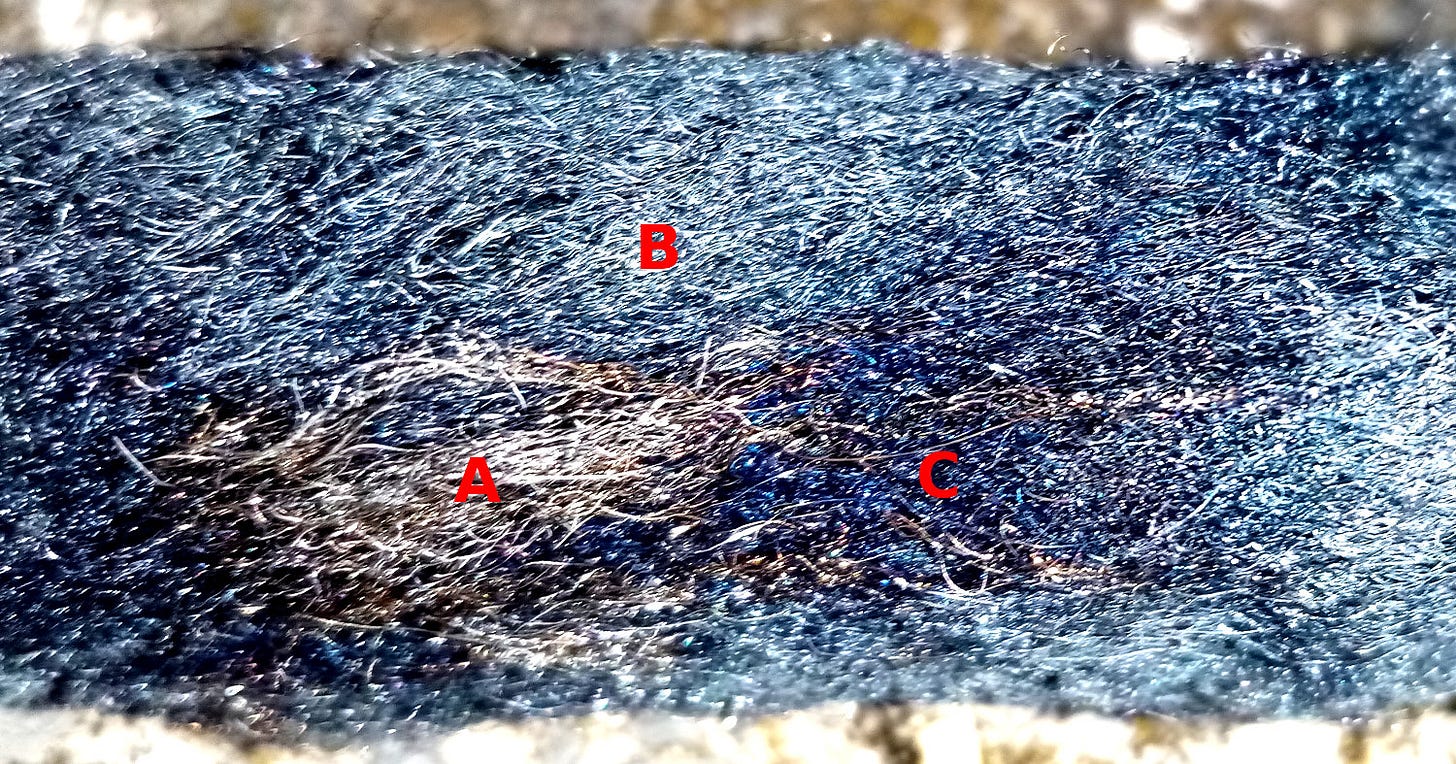
You will notice that burning of steel wool is not always complete. Some threads manage to stay away from the heat and do not burn away, or some iron in the interior of threads remains unburned. Therefore, we can make a step further and use iron powder. You can easily purchase it on the Internet. I got a powder of 90 microns grains for the next experiment.
Put some alcohol into a ceramic cup and ignite it. I use isopropanol as it burns more vigorously than ethanol. Of course, do this outside. Then throw a little bit of iron powder into the flames. The iron dust particles will immediately ignite and fly around like a firework. Actually, real fireworks are using metals to get all those vivid colors!

When your fire stops, you will notice lots of gray dust on the ground. This is mostly magnetite and you can easily find out why it got this name. Take a magnet, cover it by plastic foil, and put it over this gray dust. The dust will stick to the magnet because magnetite is magnetic! The plastic foil is very useful because getting rid of this dust from the magnet is very difficult, unless you have the foil that you can peel off. By the way, keep this magnetite dust for other STEM experiments.
The burning of iron dust is not just for a pretty entertainment. It has actual industrial application. Since burning iron dust is so easy, while it also releases lots of heat, it can be used as an efficient high-temperature energy source. The beauty here is that it does not release any gas into the atmosphere, and the produced iron oxide can be collected and transformed back into iron dust. As a part of this research on burning iron dust, scientists look at how it behaves in weightless conditions. The videos of propagating waves of burning dust are mesmerizing.