They lied to you about rainbows!
The truth will shock you! Or, how the nature of rainbows is more beautiful than textbook explanations.
On 12 November 1801, a lecture was delivered at the Royal Society of London by a brilliant young scientist who was eager to show how Newton was wrong on the theory of light. According to Newton, light consists of particles, but the lecturer disagreed. In a similar lecture, delivered a year earlier, he argued that the light is made of waves. Now, he confronted Newton’s ideas with a long list of concepts that cannot be explained using particles but can be with waves. In this lecture, some of the key working concepts of the wave nature of light were postulated for the first time. Two years later, on 24 November 1803, he gave another lecture about light being waves, but this time using experiments that secured his place in history.
The name of the lecturer was Thomas Young, and his work transformed the science of light phenomena. Today, his name stands beside that of Albert Einstein, who showed 100 years later that light is both particles and waves at the same time, which earned him the Nobel Prize. It is not surprising, then, that scientists had struggled to build a complete theory of light – it behaves like waves from the classical physics point of view, but as particles (or quanta of energy) from the quantum physics perspective (if you are highly motivated to learn more, watch this video).
Embrace the complexity
This is a very difficult concept to grasp even for physicists, who are still exploring various exotic properties of light. So, how can you approach this topic with your kids? In my view, there are three major steps we should strive to accept and implement:
a) light is anything but simple to understand: Educational materials tend to describe concepts of light phenomena as something well defined and conceptually complete. Actually, what they are showing is various mathematical and conceptual simplifications. For example, drawing a wiggly line depicts a wave, but has nothing to do with the true nature of light wave. Kids take these representations literally and misconceptions are formed in their minds.
b) pick one light phenomenon and go deep: We tend to skip from one example of light behavior to another. It is fun to watch and can motivate kids to ask for more, but if you want them to grasp a deeper conceptual knowledge, pick one phenomenon and dig dip into the details. One example, that we will discuss in the next episode, are colors of soap bubbles (do you know that many aspects of this phenomenon are still explored by scientists today?).
c) notice similar light phenomena around you: Once kids get the basic idea of concepts behind a light phenomenon, encourage them to start noticing similar things everywhere around them. Shiny rainbow colors on soap bubbles, insects, birds, crystals, and in many other situations will start to make sense. This is the goal – to help kids experience a sense of wonder at the beauty of nature.
Do not be afraid to tell kids that light is a hard topic to understand and that we can help ourselves with some simplifications that should not be taken literally. If they are aware of that distinction, they will be open to an opportunity to explore further. Start first with the home experiments that we described in our previous story on rainbows, and then move to the story that follows.
Light as waves
So, what kind of a wave are we talking about here? You have probably heard that light is an electromagnetic wave. But what does that mean?
Electric and magnetic fields exist in each point in space, everywhere around you and inside of you (one can argue that the electric and magnetic potential exist in each point, but that’s a whole new story). The question is how strong these fields are at any instance of time. If we talk about light, then the strength of these fields is oscillating at a point where the light wave is passing through. The illustration below shows this situation. It is important to understand that light comes in “packets” of waves, i.e. it is not confined to one single line. It is noticeably spread over some volume of space, which leads to quantum physics descriptions that we do not touch here.
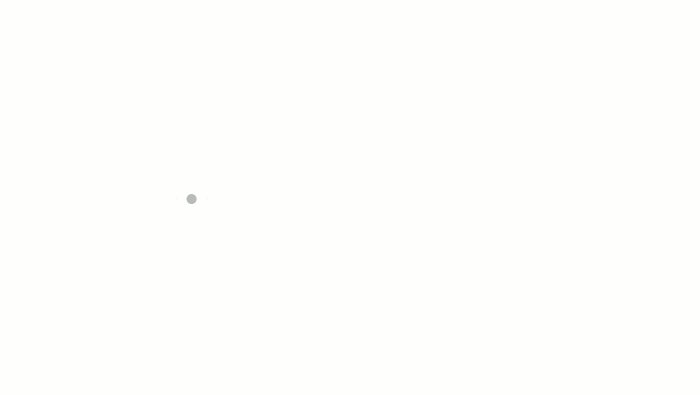
What we did not mention here is that these very same points that experience a light wave passage, are also influenced by the nearby charges that produce electric and magnetic field perturbances. So, unless we talk about a complete vacuum, the light waves interact with the surrounding material. These interactions change the light wave properties. This is why a light ray changes its direction when it crosses the boundary between two materials of different properties (i.e., air-water in case of rainbows).
A shocking truth about rainbows
In his lecture in 1801, Thomas Young introduced the principle of light interference, one of the key concepts of light as waves. Interference is a situation where interacting waves either boost or destroy their joint wave strength. See for example the graphics below. It shows two waves of electric field being added together to produce the net result. The net wave can be stronger than individual waves, or completely disappear, depending on how the two interacting waves are shifted relative to each other.
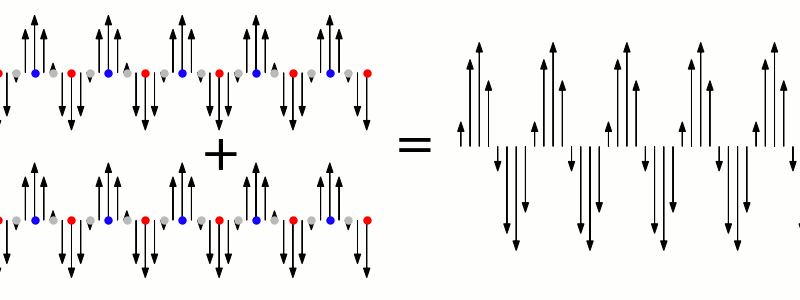
Young showed the power of light interference theory in his lecture in 1803, when he presented examples where it can be successfully applied to explain the patterns of colors. But for one of the examples related to rainbows he is not remembered, despite being correct on the light interference.
Even though the basic theory of rainbow colors formation was established already in the 17th century, it had become evident by 1800 that some rainbows display colors that do not fit into the theory. Rainbows are formed by light refraction, i.e. change of direction of light rays when they cross a boundary between air and water. Since different colors change direction by different angles, refraction of sunlight in raindrops leads to enhancements of rainbow colors in slightly different directions (more on this in our previous episode).
However, people had been noticing that below the main bow there are sometimes arcs of pastel colors, mostly of pink, purple, or green hues. These extra arcs are called supernumerary rainbows, and the refraction theory, which treated light as particles, was not able to reproduce them. Young addressed this problem and suggested that supernumerary rainbow colors are a result of the light wave interference.
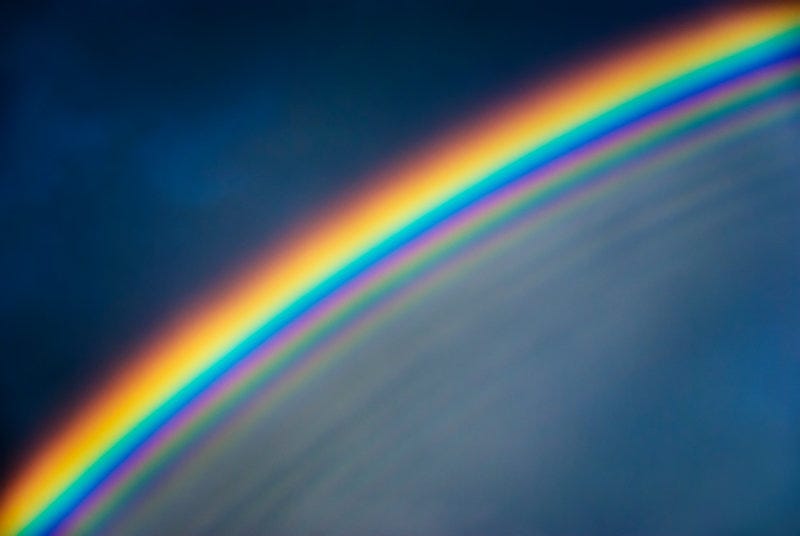
There was only one problem: the angular positions of his color maxima in supernumerary rainbow were not correct. For some reason, calculations did not match the observations.
It was the British mathematician George Biddell Airy who managed to put together an approximative calculation in 1838 that was successful in matching the data. Thomas Young was not credited for his work on light interference in supernumerary rainbows (if you are a mathematics enthusiast, read more about the Airy’s calculations here and here).
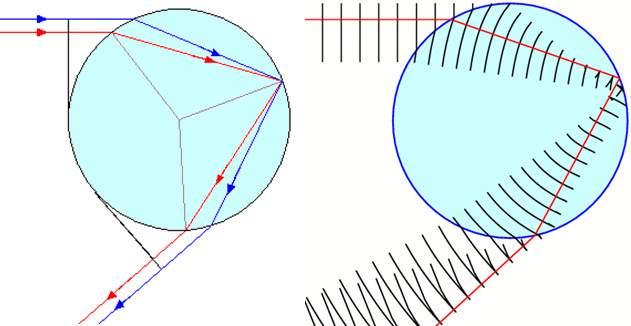
And why did Young’s approach fail? It turns out that people at that time did not know about one weird phenomenon that happens when light travels through a sphere (or a lens in general). This phenomenon is known as the Gouy phase shift, and it was described by Louis Georges Gouyin in the 1890’s. It is so weird that physicists are still working on various explanations for its existence, but it is a part of numerical routine in optics today. When included into the Young’s model of supernumerary rainbows, the results are better than the Airy’s model.
Now you are ready to see the real world
Today we have more advanced mathematical methods, combined with the computational power of computers, to find how exactly a spherical particle interacts with electromagnetic waves. The actual interaction does not happen in the approximate ways we showed here. But kids are presented with this 19th century approach, which, as you can see, is nevertheless highly complicated to grasp on the conceptual level.
Kids need to be told that interaction between light and raindrops is more complicated than these old approximations. So more complicated that understanding other types of colorful atmospheric phenomena on water droplets and ice crystals goes beyond these simplifications. For example, you have probably seen corona that forms around the sun or moon thanks to thin clouds. It is produced by the diffraction of light (another wave phenomenon) by tiny cloud droplets or small ice crystals, which leads to light interference. Even more confusing are glories, rings of colors that appear in mist or cloud when the observer is between the droplets and the sun. Their explanation is surprisingly difficult to conceptualize even today, even though the modern theory gives the correct predictions (for more rigorous discussion for physics enthusiasts see here).
In the next story we will move to the next level of rainbow magic – finding rainbows everywhere around us. And we will make a chocolate that shines like a rainbow.